Table of Contents
Biomedical importance
Water is the predominant chemical component of living organisms. Its unique physical properties, which include the ability to solvate a wide range of organic and inorganic molecules, derive from water’s dipolar structure and exceptional capacity for forming hydrogen bonds. How water interacts with solvated biomolecules influences the structure both of the biomolecule and of water itself. An excellent nucleophile, water is a reactant or product in many metabolic reactions. Regulation of water balance depends upon hypothalamic mechanisms that control thirst, on antidiuretic hormone (ADH), on retention or excretion of water by the kidneys, and on evaporative loss. Nephrogenic diabetes insipidus, which involves the inability to concentrate urine or adjust to subtle changes in extracellular fluid osmolarity, results from the unresponsiveness of renal tubular osmoreceptors to ADH. Water has a slight propensity to dissociate into hydroxide ions and protons. The concentration of protons, or acidity, of aqueous solutions, is generally reported using the logarithmic scale. Bicarbonate and other buffers normally maintain the pH of extracellular fluid between 7.35 and 7.45. Suspecteddisturbances of acid-base balance are verified by measuring the pH of arterial blood and the CO2 content of venous blood.Causes of acidosis (blood pH <7.35) include diabetic ketosisand lactic acidosis. Alkalosis (pH >7.45) may follow vomiting of acidic gastric contents.
Water is an ideal biologic solvent
Water Molecules Form Dipoles
A water molecule is an irregular, slightly skewed tetrahedron with oxygen at its center (Figure 2–1). The two hydrogens and the unshared electrons of the remaining two sp3-hybridized orbitals occupy the corners of the tetrahedron. The 105° angle between the two hydrogen atoms differs slightly from the ideal tetrahedral angle, 109.5°. Ammonia is also tetrahedral, with a107° angle between its three hydrogens. The strongly electronegative oxygen atom in a water molecule attracts electrons away from the hydrogen nuclei, leaving them with a partial positive charge, while its two unshared electron pairs constitute a region of local negative charge.

A molecule with an electrical charge distributed asymmetrically about its structure is referred to as a dipole. Water’sstrong dipole is responsible for its high dielectric constant. As described quantitatively by Coulomb’s law, the strength of interaction F between oppositely charged particles is inversely proportionate to the dielectric constant ε of the surrounding medium. The dielectric constant for a vacuum is essentially unity; for hexane, it is 1.9; for ethanol, 24.3; and for water at 25°C, 78.5. Water therefore greatly decreases the force of attraction between charged and polar species relative to water-free environments with lower dielectric constants. Its strong dipole and high dielectric constant enable water to dissolve large quantities of charged compounds such as salts.
Water Molecules Form Hydrogen Bonds
A partially unshielded hydrogen nucleus covalently bound to electron-withdrawing oxygen or a nitrogen atom can interact with an unshared electron pair on another oxygen or nitrogen atom to form a hydrogen bond. Since water molecules contain both of these features, hydrogen bonding favors the self-association of water molecules into ordered arrays(Figure 2–2). Hydrogen bonding profoundly influences the physical properties of water and accounts for its relatively high viscosity, surface tension, and boiling point. On average, each molecule in liquid water associates hydrogen bonds with 3.5 others. These bonds are both relatively weak and transient, with a half-life of a few picoseconds. Rupture of a hydrogen bond in liquid water requires only about 4.5 kcal/mol, less than 5% of the energy required to rupture a covalent O—H bond.

Hydrogen bonding enables water to dissolve many organic biomolecules that contain functional groups which can participate in hydrogen bonding. The oxygen atoms of aldehydes, ketones, and amides, for example, provide lone pairs of electrons that can serve as hydrogen acceptors. Alcohols, carboxylic acids, and amines can serve both as hydrogen acceptors and as donors of unshielded hydrogen atoms for the formation of hydrogen bonds (Figure 2–3).

bonding (Figure 2-3)
Interaction with water influences the structure of biomolecule
Covalent and Noncovalent Bonds Stabilize Biologic Molecules
The covalent bond is the strongest force that holds molecules together (Table 2–1). Noncovalent forces, while of lesser magnitude, make significant contributions to the structure, stability, and functional competence of macromolecules in living cells. These forces, which can be either attractive or repulsive, involve interactions both within the biomolecule and between and the water that forms the principal component of the surrounding environment.
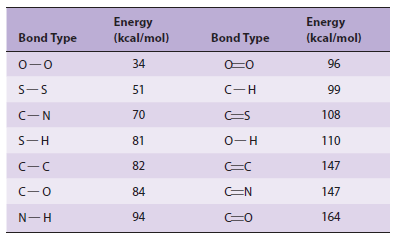
Significance (Table 2-1)
Biomolecules Fold to Position Polar Charged Groups on Their Surfaces
Most biomolecules are amphipathic; that is, they possess regions rich in charged or polar functional groups as well as regions with hydrophobic character. Proteins tend to fold with the R-groups of amino acids with hydrophobic side chains in the interior. Amino acids with charged or polar amino acid side chains (eg, arginine, glutamate, serine, generally are present on the surface in contact with water. A similar pattern prevails in a phospholipid bilayer where the charged “head groups” of phosphatidylserine or phosphatidylethanolamine contact water while their hydrophobic fatty acyl side chains cluster together, excluding water. This pattern maximizes the opportunities for the formation of energetically favorable charge-dipole,dipole-dipole, and hydrogen bonding interactions between polar groups on the biomolecule and water. It also minimizes energetically unfavorable contacts between water and hydrophobic groups.
Hydrophobic Interactions
Hydrophobic interaction refers to the tendency of nonpolar compounds to self-associate in an aqueous environment. This self-association is driven neither by mutual attraction nor by what is sometimes incorrectly referred to as “hydrophobic bonds.” Self-association minimizes the disruption of energetically favorable interactions between the surrounding water molecules. While the hydrogens of nonpolar groups such as the methylene groups of hydrocarbons do not form hydrogen bonds, they do affect the structure of the water that surrounds them. Water molecules adjacent to a hydrophobic group are restricted in the number of orientations (degrees of freedom)that permit them to participate in the maximum number of energetically favorable hydrogen bonds. Maximal formation of multiple hydrogen bonds, which maximizes enthalpy, can be maintained only by increasing the order of the adjacent water molecules, with an accompanying decrease in entropy. It follows from the second law of thermodynamics that the optimal free energy of a hydrocarbon-water mixture is a function of both maximal enthalpy (from hydrogen bonding)and highest entropy (maximum degrees of freedom). Thus, nonpolar molecules tend to form droplets that minimize exposed surface area and reduce the number of water molecules whose emotional freedom becomes restricted. Similarly, in the aqueous environment of the living cell the hydrophobic portions of biopolymers tend to be buried inside the structure of the molecule, or within a lipid bilayer, minimizing contact with water.
Electrostatic Interactions
Interactions between charged groups help shape biomolecular structure. Electrostatic interactions between oppositely charged groups within or between biomolecules are termed salt bridges. Salt bridges are comparable in strength to hydrogen bonds but act over larger distances. They, therefore, often facilitate the binding of charged molecules and ions to proteins and nucleic acids.
van der Waals Forces
van der Waals forces arise from attractions between transient dipoles generated by the rapid movement of electrons of all neutral atoms. Significantly weaker than hydrogen bonds but potentially extremely numerous, van der Waals forces decrease as the sixth power of the distance separating atoms (Figure 2–4). Thus, they act over very short distances, typically 2 to 4 A.

with the distance, R, between interacting species (Figure 2–4)
Multiple Forces Stabilize Biomolecules
The DNA double helix illustrates the contribution of multiple forces to the structure of biomolecules. While each DNA strand is held together by covalent bonds, the two strands of the helix are held together exclusively by noncovalent interactions such as hydrogen bonds between nucleotide bases (Watson-Crick base pairing) and van der Waals interactions between the stacked purine and pyrimidine bases. The double helix presents the charged phosphate groups and polar hydroxyl groups from the ribose sugars of the DNA backbone to water while burying the relatively hydrophobic nucleotide bases inside. The extended backbone maximizes the distance between negatively charged phosphates, minimizing unfavorable electrostatic interactions.